Recommendations
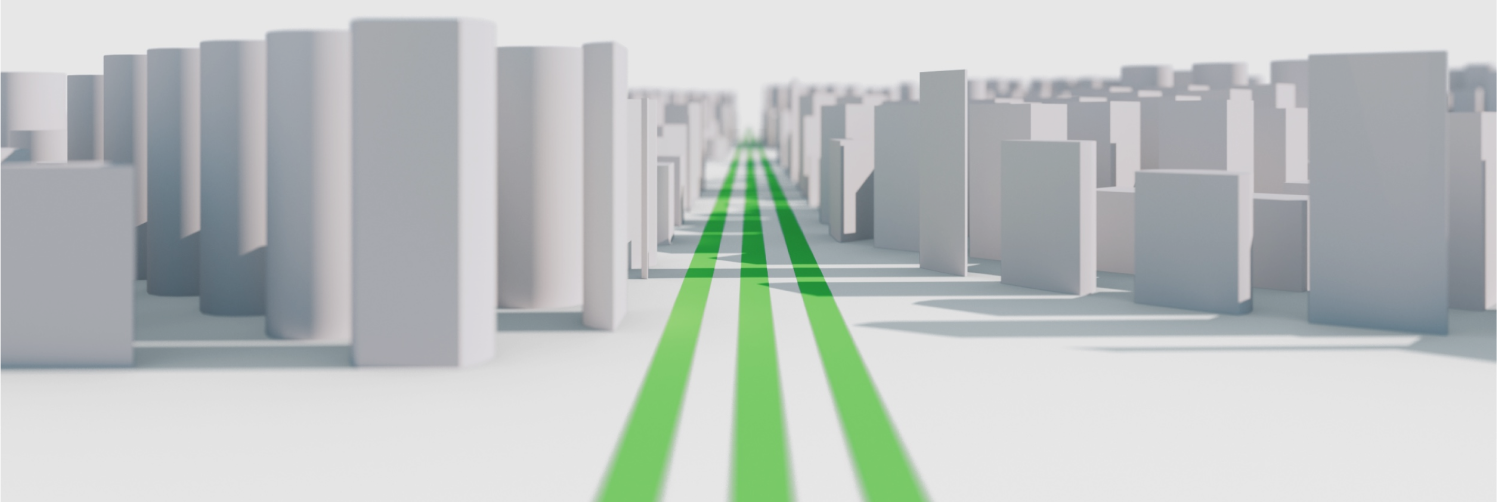
Our recommendations in a nutshell
Under all three scenarios, electrification leads to a one-third reduction in Belgium’s total energy consumption by 2050 – down to 250 TWh. At the same time, electricity demand at least doubles to 155 TWh, mainly due to the electrification of buildings, road transport and industry. Meeting this growing electricity demand will thus require significant investments in wind energy and electricity infrastructure, regardless of which pathway is chosen.
Across all three scenarios, we therefore propose the following short-, mid- and long-term recommendations.
Short-term recommendations: what do we need to do between now and 2030?
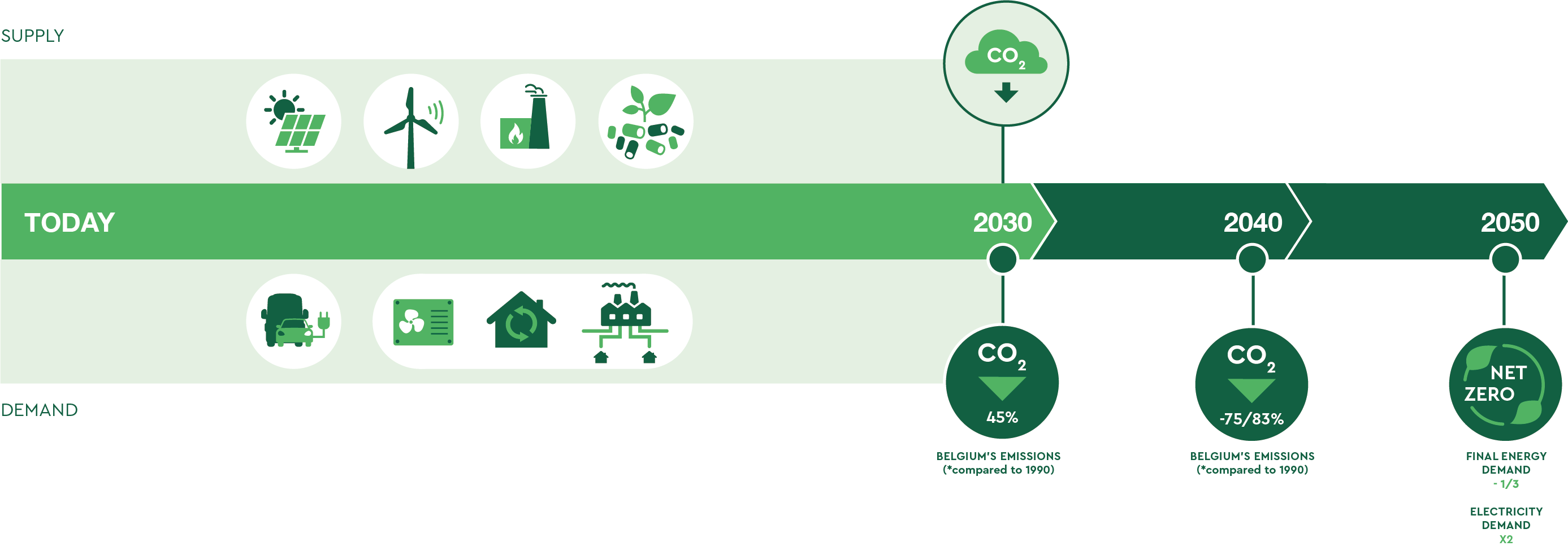
Cut Belgium's emissions by 45% compared to 1990 levels by:
Accelerating the electrification of road transport and buildings by speeding up the rollout of electric vehicles and heat pumps - combined with a strong acceleration of the renovation of our buildings.
Scaling up wind and solar power generation, and doing so rapidly.
Banning new fossil heating systems in buildings and expand district heating networks.
Investing in new gas-fired power plants to support electricity production.
Defining a clear policy framework for CO2 storage projects such as Kairos@C, Go4Zero and H2BE to enable upscaling by 2040. Starting to build a CO2 backbone.
Setting up a strategic plan as soon as possible if the decision is made to introduce new nuclear energy by 2040–2045.
- Defining a clear policy framework for hydrogen (H2), based on technological neutrality.
Mid-term recommendations: what do we need to do between 2030 and 2040?
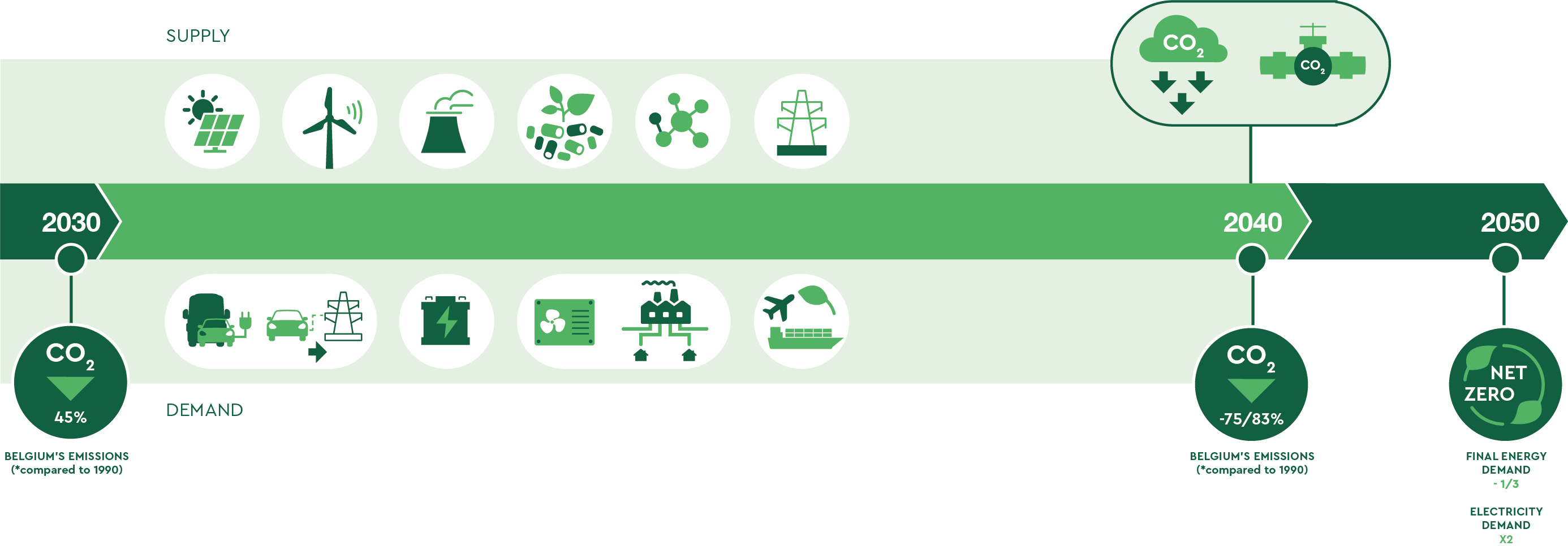
Cut Belgium's emissions by 75%-83% compared to 1990 levels by:
Ensuring that at least 50% of non-residential and 80% of residential buildings use heat pumps or district heating.
Electrifying nearly all road transport.
Securing access to additional offshore wind capacity beyond the Belgian North Sea.
Deploying large-scale battery systems and enable vehicle-to-grid use for daily storage and self-consumption.
Defining a clear policy framework for the import of hydrogen and derivatives, and synthetic fuels.
Building CO₂ pipeline infrastructures to allow for carbon transport and storage.
Long-term recommendations: what do we need to do between 2040 and 2050?
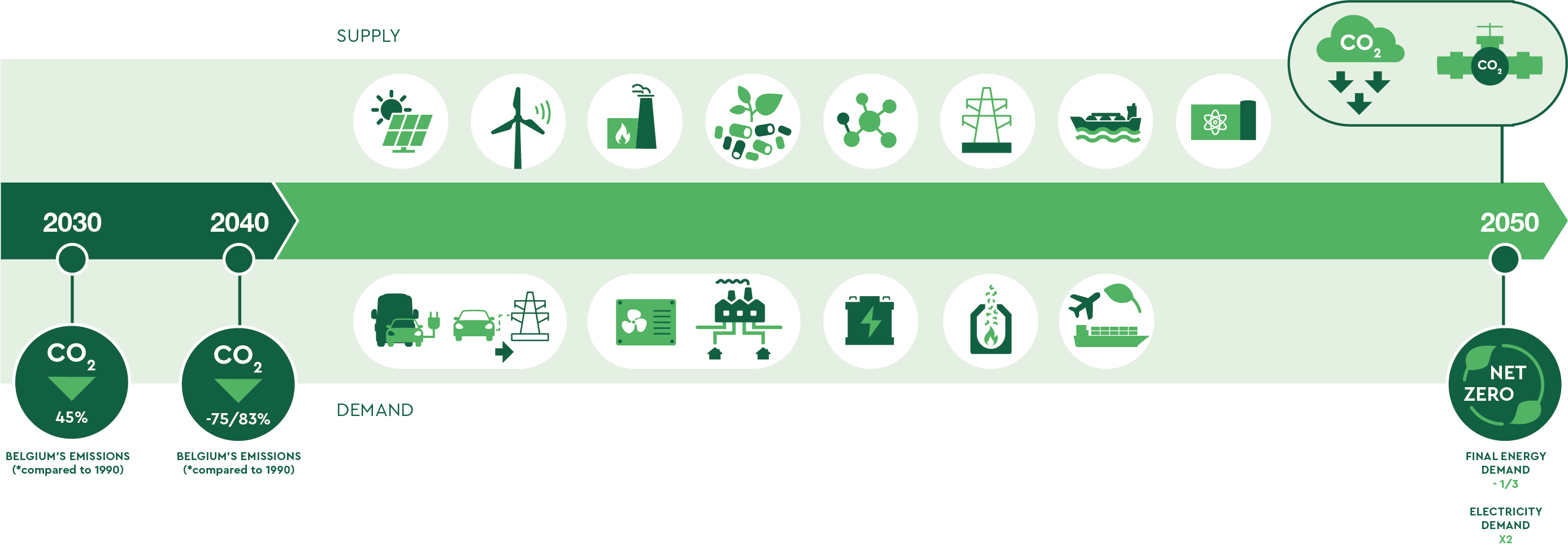
Reach full climate neutrality by:
Switching entirely to climate neutral energy sources in both buildings and transport.
Developing flexible nuclear capacity that complements variable renewable energy, f.e. by producing hydrogen when there is a surplus.
Collaborating internationally to import hydrogen carriers and synthetic fuels such as ammonia, e-methane, methanol and e-kerosene, which are crucial for sectors such as international aviation and shipping.
Capture and store carbon emissions from energy-intensive industry.
Our recommendations in full detail
Short-term recommendations: what do we need between now and 2030?
Belgium can reduce its emissions by 45% by 2030 compared to 1990 levels, according to the TIMES-Be model used in this study.
The model shows that both high CO₂ prices (185€/ton) and – interestingly – even lowerCO₂ prices (80€/ton) in 2030 lead to comparable emission reductions, because the system anticipates the need to reach net-zero by 2050 and reacts to increasing CO₂ prices over time.
However, in a sensitivity scenario where CO₂ prices remain lower (80€/ton by 2030) and no long-term climate neutrality target is assumed, the outcome is very different: industrial emissions barely decline, and the total emissions reduction by 2030 drops to just 37%. This underlines the importance of maintaining a clear long-term decarbonisation trajectory.
By 2022, sectors under the EU Emissions Trading System (ETS) had already reduced emissions by 38%, while non-ETS sectors had only achieved a 7% reduction. This leaves a significant remaining gap of 36 percentage points to close in the non-ETS sectors by 2030.
Changing how we use energy
Transport | Electrification is key
Rapid electrification of road transport plays a crucial role in reducing emissions. By 2030, nearly 2 million fully electric vehicles are expected on the road. To achieve this, annual EV sales must double within the next three years, targeting a 60% market share. This electrification trend is consistent with the requirements of the RED III regulation for transport.
Buildings | Renovation, heat pumps and district heating
In both residential and commercial buildings, a strong increase in insulation is needed before 2040. This requires immediate action. In the residential sector, the model assumes a 4% annual renovation rate, leading to a 50% reduction in heat demand in 20% of the current building stock by 2030, especially in homes with energy label D or lower.
The model finds that no new fossil-based heating systems are cost-optimal, neither in residential nor in commercial buildings. As a result, demand is progressively electrified. Replacing fossil heating systems requires the same 4% annual rate, which would result in around 30% of homes using heat pumps or district heating by 2030. This transformation represents a greater effort than insulation, as it is only just beginning. Ideally – according to the model, which does not account for system inertia –this share would reach 43% by 2030 (36% via electric heat pumps and 7% via district heating), compared to less than 10% today.
Industry | Electrification of low-temperature processes
In the industrial sector, electrification is applied to low-temperature heat demand, notably in the food, pulp, paper and wood sectors, which switch to heat pumps.
Transforming how we produce energy
Wind and solar energy | The need for speed
To meet growing electricity demand, renewable energy generation must expand significantly.
Offshore wind capacity should increase rapidly, both in the Belgian North Sea and through direct connections to far offshore zones. The model finds it cost-effective to have access to more than 7 and up to 9.3 GW of offshore wind capacity by or shortly after 2030. This highlights the urgency of establishing a cross-border framework to accelerate the realisation of existing offshore ambitions.
For solar PV, the model indicates a need to maintain an installation rate of more than 1 GW per year from now onwards, to reach 17–19 GW of capacity by 2030. The model does not provide insights into grid balancing, capacity needs or storage due to its annual time resolution. Therefore, additional studies are needed on the integration of large volumes of PV and storage solutions.
Thermal generation | Securing backup capacity
New thermal capacity – including natural gas-based CHP (combined heat and power) and combined-cycle gas turbines (CCGTs) – will be needed to ensure electricity supply during periods of low renewable generation. Total capacity may reach 9-10 GW. These plants will operate most intensively between 2025 and 2030, compensating for reduced nuclear capacity. Newer, high-efficiency units may run for more than 4,500 hours annually in this period.
Nuclear energy | Deciding on lifetime extension and planning ahead
To extend the operation of 2 GW of existing nuclear capacity (Doel 4 and Tihange 3) by 20 years, a decision is needed by 2030. If Belgium intends to deploy new nuclear capacity by 2040–2045, a long-term framework must be designed. This includes clear commitments related to capital, human capital, public and private sector responsibilities, locations and permits.
Carbon Capture and Storage | Laying the foundation
To enable the first CCS projects in Belgium by 2030 – and to allow for significant expansion by 2040 – a clear CCS policy framework must be put in place. By 2030, CO₂ capture volumes of 1.5 to 4 million tonnes could be achieved through projects such as Kairos@C, Go4Zero, H2BE and others.
Belgium can reduce its emissions by 45% by 2030 compared to 1990 levels, according to the TIMES-Be model used in this study.
The model shows that both high CO₂ prices (185€/ton) and – interestingly – even lowerCO₂ prices (80€/ton) in 2030 lead to comparable emission reductions, because the system anticipates the need to reach net-zero by 2050 and reacts to increasing CO₂ prices over time.
However, in a sensitivity scenario where CO₂ prices remain lower (80€/ton by 2030) and no long-term climate neutrality target is assumed, the outcome is very different: industrial emissions barely decline, and the total emissions reduction by 2030 drops to just 37%. This underlines the importance of maintaining a clear long-term decarbonisation trajectory.
By 2022, sectors under the EU Emissions Trading System (ETS) had already reduced emissions by 38%, while non-ETS sectors had only achieved a 7% reduction. This leaves a significant remaining gap of 36 percentage points to close in the non-ETS sectors by 2030.
Changing how we use energy
Transport | Electrification is key
Rapid electrification of road transport plays a crucial role in reducing emissions. By 2030, nearly 2 million fully electric vehicles are expected on the road. To achieve this, annual EV sales must double within the next three years, targeting a 60% market share. This electrification trend is consistent with the requirements of the RED III regulation for transport.
Buildings | Renovation, heat pumps and district heating
In both residential and commercial buildings, a strong increase in insulation is needed before 2040. This requires immediate action. In the residential sector, the model assumes a 4% annual renovation rate, leading to a 50% reduction in heat demand in 20% of the current building stock by 2030, especially in homes with energy label D or lower.
The model finds that no new fossil-based heating systems are cost-optimal, neither in residential nor in commercial buildings. As a result, demand is progressively electrified. Replacing fossil heating systems requires the same 4% annual rate, which would result in around 30% of homes using heat pumps or district heating by 2030. This transformation represents a greater effort than insulation, as it is only just beginning. Ideally – according to the model, which does not account for system inertia –this share would reach 43% by 2030 (36% via electric heat pumps and 7% via district heating), compared to less than 10% today.
Industry | Electrification of low-temperature processes
In the industrial sector, electrification is applied to low-temperature heat demand, notably in the food, pulp, paper and wood sectors, which switch to heat pumps.
Transforming how we produce energy
Wind and solar energy | The need for speed
To meet growing electricity demand, renewable energy generation must expand significantly.
Offshore wind capacity should increase rapidly, both in the Belgian North Sea and through direct connections to far offshore zones. The model finds it cost-effective to have access to more than 7 and up to 9.3 GW of offshore wind capacity by or shortly after 2030. This highlights the urgency of establishing a cross-border framework to accelerate the realisation of existing offshore ambitions.
For solar PV, the model indicates a need to maintain an installation rate of more than 1 GW per year from now onwards, to reach 17–19 GW of capacity by 2030. The model does not provide insights into grid balancing, capacity needs or storage due to its annual time resolution. Therefore, additional studies are needed on the integration of large volumes of PV and storage solutions.
Thermal generation | Securing backup capacity
New thermal capacity – including natural gas-based CHP (combined heat and power) and combined-cycle gas turbines (CCGTs) – will be needed to ensure electricity supply during periods of low renewable generation. Total capacity may reach 9-10 GW. These plants will operate most intensively between 2025 and 2030, compensating for reduced nuclear capacity. Newer, high-efficiency units may run for more than 4,500 hours annually in this period.
Nuclear energy | Deciding on lifetime extension and planning ahead
To extend the operation of 2 GW of existing nuclear capacity (Doel 4 and Tihange 3) by 20 years, a decision is needed by 2030. If Belgium intends to deploy new nuclear capacity by 2040–2045, a long-term framework must be designed. This includes clear commitments related to capital, human capital, public and private sector responsibilities, locations and permits.
Carbon Capture and Storage | Laying the foundation
To enable the first CCS projects in Belgium by 2030 – and to allow for significant expansion by 2040 – a clear CCS policy framework must be put in place. By 2030, CO₂ capture volumes of 1.5 to 4 million tonnes could be achieved through projects such as Kairos@C, Go4Zero, H2BE and others.
Mid-term recommendations: what do we need between 2030 and 2040?
CO2 emissions should decrease by 75-83% compared to Belgium’s 1990 emissions, to be on track for achieving 2050 net-zero.
Evolving energy demand
Transport: Widespread electrification and new fuels
By 2040, road transport is almost fully electrified across all modelled scenarios, confirming it’s the cost-effectiveness of electrification as a no-regret measure. Unlocking the flexibility potential of vehicle-to-grid applications will require early coordination among policymakers, grid operators, vehicle manufacturers, charging infrastructure providers, and end users—starting in the early 2030s.
In international transport:
• Aviation will need to use at least 34% sustainable aviation fuels (SAFs), amounting to 3 TWh of fuel demand for Belgium.
• The maritime sector must reduce carbon intensity by 31%. Compliance with the legislation can be achieved through the use of dual-fuel LNG engines, which are 30% more efficient and have a 24% lower emission factor than traditional heavy fuel oil.
• In the IMPORTS scenario, access to cheaper green ammonia leads to an uptake of 2 TWh as maritime fuel by 2040.
Buildings: Deep renovation and fossil phase-out
Efforts in the buildings sector—both residential and commercial— must remain just as high after 2030 as before. In the residential sector, maintaining a 4% annual renovation rate would result in a 50% reduction in heat demand across 60% of the current building stock. At the same time, phasing out fossil heating at a similar 4% would lead to around 70% of homes using heat pumps or district heating by 2040.
In densely populated areas, district heating networks will expand, delivering up to 11.5 TWh of heat from sources such as waste incineration, deep geothermal energy, and biomass-fueled CHP. By 2040, oil heating will be nearly phased out, with less than 4 TWh of demand remaining.
Expanding and balancing the energy supply
Electricity system: Renewables, flexibility and imports
Total renewable electricity capacity grows to at least 44 GW, with major investments in:
• Offshore wind: 11–16 GW
• Onshore wind: 8–10 GW
• Solar PV: In scenarios with less offshore wind and/or nuclear capacity (REACTORS and IMPORTS), PV capacity increases to 20–29 GW
To maintain system stability, batteries and vehicle-to-grid solutions play a key role in day-night storage and flexibility:
• Battery storage reaches 1.3–2.5 GW by 2040, in line with the temporal detail the TIMES-Be model captures. This reflects the minimum needs, and actual needs may be higher when accounting for different weather years and using more detailed temporal modeling, including grid balancing and ancillary services.
• Vehicle-to-grid is providing 0.5–0.7 GW of storage/flexibility.
In the IMPORTS scenario, 1 GW of additional interconnection capacity is needed by 2040, enabling up to 25 TWh of net electricity import.
Nuclear energy: Waiting for small/advanced modular REACTORS
The TIMES-Be model does not invest in large scale, non-flexible nuclear capacity (EPR Gen III+) based on least-cost optimisation, but decides to wait for small/advanced modular REACTORS to appear by 2045.
To test the impact of new nuclear, a sensitivity analysis includes a forced investment in one 1.6 GW large non-flexible reactor from 2040 onwards. This leads to similar flexibility needs–gas turbines and 2.5 GW of battery storage–as the IMPORTS scenario without new nuclear. The analysis shows that if SMRs are available and cost-competitive by 2045, there may be no added value in investing in a large EPR Gen III+ reactor–either from an energy system perspective or in terms of cost-effectiveness. However, in a sensitivity analysis where SMRs are not available, the EPR Gen III+ is selected.
Carbon Capture and Storage: Scaling up fast
Between 2030 and 2040, CCS expands significantly. Total CO2 captured, transported and stored reaches 20 million tonnes per year by 2040. CCS infrastructure becomes essential to connect industrial sites with hard-to-abate process emissions in sectors such as cement, lime, glass, high value chemicals and iron & steel.
In the ROTORS scenario, where Belgium has a limited access to CO2 storage, captured volumes are lower–6.5 million tonnes per year–primarily from cement, lime and glass. This scenario results in the highest remaining CO2 emissions in Belgium across all scenarios: 30 million tonnes per year by 2040.
Long-term recommendations: what do we need between 2040 and 2050?
All modelled scenarios reach net-zero emissions by 2050, with 2 million tonnes of CO2 emissions remaining. This outcome results from an exogenous constraint on emissions imposed on the model.
Transforming energy demand
Buildings and road transport: Full electrification achieved
By 2050, buildings and road transport–including both the passenger fleet and trucks– are fully electrified, except for 13 TWh of district heating. If more biomethane were available, heating buildings in large cities could be achieved without the need to roll out district heating networks.
Industry: Second wave of electrification
From 2045 onwards, a second wave of industrial electrification occurs. In the ROTORS scenario, stable and affordable electricity enables the electrification of processes such as the naphta cracker furnaces, and specific iron & steel productions steps, including Electric Arc Furnaces for DRI and the electrification of casting and rolling.
Future energy supply: Balancing renewables, molecules and flexibility
Wind and solar energy: Steady growth to meet rising electricity demand
By 2050, total electricity demand as final energy increases from 155 to 170 TWh, depending on the scenario–IMPORTS being the lowest, ROTORS the highest. To meet this growing demand, the model shows a continuous expansion of wind and solar PV capacity, reaching 65 to 80 GW of renewable electricity capacity by 2050.
To ensure balance and flexibility in the system:
• Battery storage capacity increases to 6–7.3 GW
• Vehicle-to-grid services contribute more than 2.5 GW of flexibility
In the IMPORTS scenario, interconnection capacity expands to over 8 GW by 2050 (peak import capacity, excluding transit flows), enabling up to 32 TWh of net electricity import.
Thermal generation: Bio-based and clean molecule integration
By 2050, thermal capacity (non-nuclear) remains relatively stable at 7.5 to 9.3 GW across scenarios. Within that total:
• 1.8 to 2.6 GW is biobased, operating more than 3,000 hours annually
• The remaining gas-based capacity switches from natural gas to clean molecules between 2045 and 2050–driven by the target to reach net-zero–operating a maximum of 1,000 hours per year
Nuclear energy: New capacity in selected scenarios
In the ROTORS and REACTORS scenarios, new nuclear capacity of 4 to 8 GW respectively is included as part of the energy system after 2040. The REACTORS can operate up to 7,000 full-load hours annually, while also providing flexibility when needed.
In these scenarios, nuclear generation is combined with a Solid Oxide Electrolysers, which can produce up to 16 TWh of hydrogen. This hydrogen may be combined with captured CO2 in methanol synthesis, serving as feedstock for plastics production through methanol-to-olefins.
Clean molecules: Demand driven by international transport
The defossilisation targets of international transport–especially maritime shipping and aviation– drives a large demand for clean molecules. The model assumes a full shift to net-zero in international shipping and a 70% reduction in emissions from international aviation, in line with EU regulations.
As a result:
• Maritime transport requires 44 to 59 TWh of imported e-methane and ammonia by 2050
• Aviation uses up to 15 TWh of e-kerosene
The power sector shifts from using natural gas in the thermal plant to hydrogen, hydrogen-based derivatives, and e-methane by 2050, from a mere 4 TWh to almost 20 TWh in the IMPORTS scenario.
To unlock large-scale import of green hydrogen derivatives into the EU, coordinated action is needed across policy frameworks, strategic port and pipeline infrastructure, and resilient supply chain market dimensions with clear certification, risk-sharing, and digital tracking systems.
Carbon Capture and Storage: Key for hard-to-abate sectors
CCS remains critical to reduce around 20 million tonnes of CO2 from hard-to-abate industrial processes and residual fossil energy use. While fossil fuel use in industry declines by 36 to 54 %, it still ranges between 36 to 50 TWh depending on the scenario.
In the ROTORS scenario, the model captures 13.7 million tonnes of CO2, even though only 10 million tonnes can be stored annually. The remaining 3.7 million tonnes is used for methanol production, as described above.