Molecules
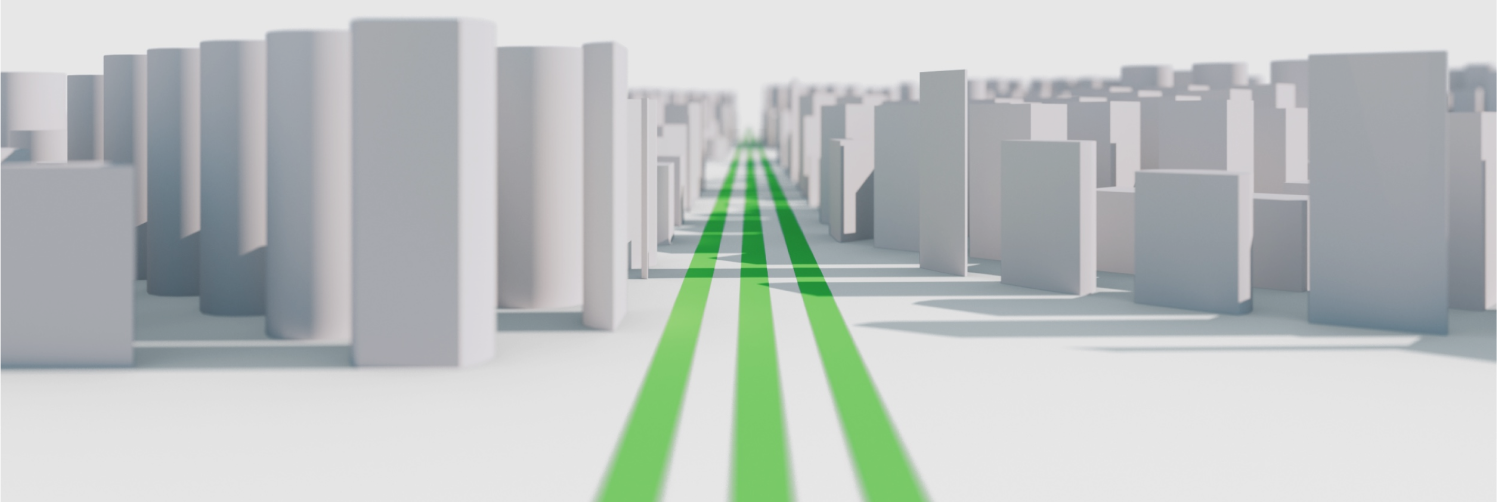
Molecules will be essential in a net-zero energy system
On this page, we present the scenario results related to the use of 'molecules' as energy sources or carriers in Belgium’s future energy system. The term 'molecules' serves as a collective label for a diverse set of energy carriers, which we have clustered into two main categories for reporting purposes:
Methane: including natural gas and bio-methane
Hydrogen and its derivatives: such as ammonia, synthetic methane, methanol, and kerosene
The role of methane (CH4) changes towards a net-zero energy system, but remains important
Methane—primarily in the form of natural gas—is currently the dominant fossil-based energy source, with a total use of over 100 TWh in final energy demand. By 2050, this figure is expected to decline significantly to between 21 and 35 TWh, depending on the scenario pathway.
As natural gas use phases out in applications such as low-temperature heating for buildings and industry, it remains in use for specific high-temperature processes, particularly in the iron & steel, chemical, and non-metallic minerals sectors. In these cases, its continued use is closely tied to the availability of Carbon Capture and Storage (CCS). Scenarios that assume unlimited access to CO₂ storage tend to retain more natural gas in the system.
In the power sector, natural gas use is projected to peak around 2030, reaching 42 to over 50 TWh, depending on the scenario. After that, its role diminishes, with gas turbines operating primarily as peak plants with low annual operating hours by 2050. Over time, natural gas is gradually replaced by imported e-methane (3–8 TWh) and other hydrogen-based derivatives. Notably, e-methane could play a major role in international maritime transport, where it serves as a key decarbonized fuel option.
By 2050, the energy system is expected to incorporate 29–43 TWh of biomass and biomethane, including domestic production and imports. Biomethane is mainly allocated to the maritime sector, where it fuels dual-fuel engines and helps offset the higher costs of e-methane. Meanwhile, solid biomass is primarily used in industry and in combined heat and power (CHP) plants.
Importantly, when biomass is combined with CCS in industrial processes, it can result in net-negative emissions—a benefit that would be lost if biomass were instead converted into liquid biofuels for road or aviation use.
Hydrogen and derivatives
Demand
Clean molecules—including hydrogen and its derivatives such as synthetic methane, methanol, ammonia, and synthetic fuels—will become increasingly important in a net-zero energy system. Despite this, their role in final energy demand remains limited due to the model’s least-cost optimization, which generally favors electrification as the more cost-effective decarbonization route.
However, upcoming EU legislation, like RED III, FuelEU Maritime, ReFuelEU Aviation, and the extended ETS, is expected to drive demand for sustainable fuels, particularly in hard-to-electrify sectors. For example: International aviation must reach 70% sustainable fuels by 2050 and Maritime transport is mandated to achieve net-zero emissions by 2050 (see Transport Sector Page).
Meeting these targets will require the import of large volumes of clean molecules, reaching at least 57 TWh across all scenario pathways, primarily to serve these international transport modes. Additionally, clean molecules remain essential for specific applications such as industrial feedstocks, residual refinery processes, and niche final energy demands where electrification is not feasible.
In the power sector, the demand for clean molecules varies significantly by scenario:
In ROTORS and REACTORS, demand remains relatively low—around 4 TWh by 2050;
In the IMPORTS scenario, demand rises to nearly 20 TWh, driven by limited domestic renewables (e.g., offshore wind) and the absence of new nuclear capacity. In this case, low-cost imports of clean molecules are used to fuel gas turbines during periods of electricity scarcity, making them a vital flexibility solution.
Supply
Belgium’s supply of clean molecules is expected to rely heavily on imports, particularly to meet the growing demand for decarbonized international transport fuels. By 2050, at least 69 TWh of clean molecules, including e-methane, ammonia, and e-kerosene, will need to be imported. In the IMPORTS scenario, where global clean molecule prices are lower, this volume could rise to over 100 TWh, reflecting a deeper dependence on international supply chains.
Domestic production of green hydrogen via electrolysis powered by renewable energy is expected to be negligible, due to limited cost competitiveness. However, under certain conditions—such as those in the ROTORS scenario, which assumes ample access to affordable electricity but limited CO₂ storage—the domestic production of e-hydrocarbons may become economically viable. This production goes hand in hand with the deployment of nuclear Small Modular Reactors (SMRs). Nuclear technology could enable high-temperature Solid Oxide Electrolysis (SOE), an efficient process for hydrogen production. In such a setup, 1 to 2 GW (REACTORS-ROTORS) of nuclear capacity could produce 7–16 TWh of hydrogen by 2050 with almost full load electrolyzer operation. Combined with captured CO₂ this could be used in domestic molecule synthesis processes.
In addition, hydrogen is also produced as a by-product in industrial processes such as refining and chlorine production, contributing up to 6 TWh to the national hydrogen supply.