Main Edition 2025
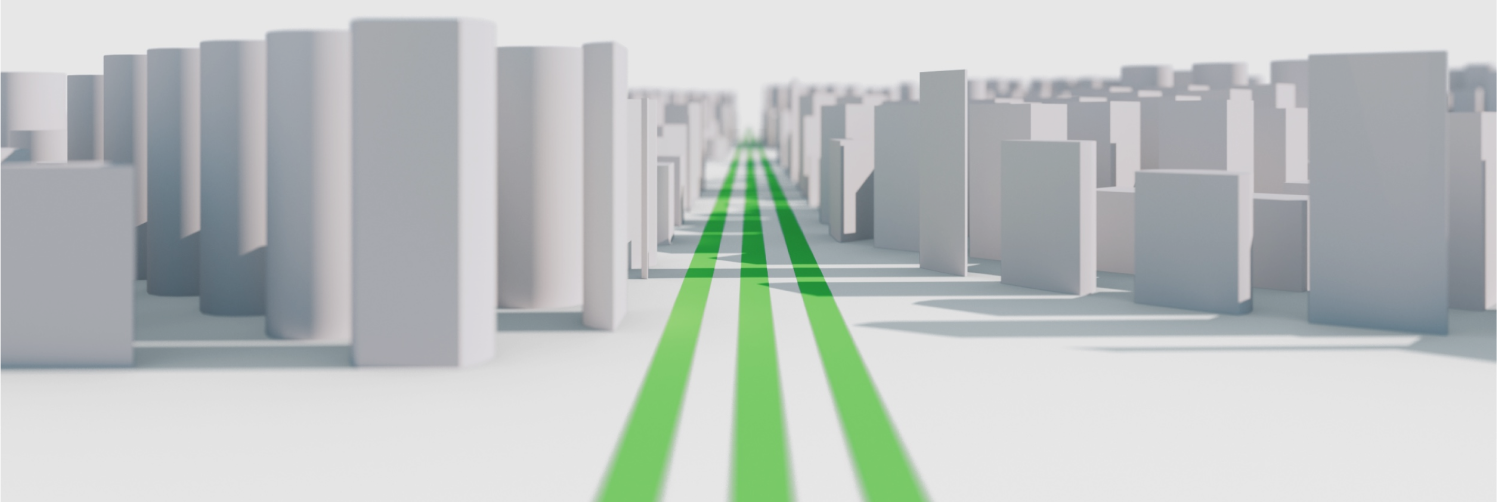
The PATHS2050 Coalition
By bringing together key Belgian industry players to exchange and challenge their insights on the future energy system, we lay the foundation for robust pathways to net-zero emissions by 2050.
In the beginning of 2024, VITO at EnergyVille joined forces with five key industry partners, active in electricity supply, and electricity and gas transmission to industry demand. Together with these PATHS2050 Coalition Partners – Arcelor-Mittal, BASF, Elia, Fluxys and Luminus – we have since then been able to exchange and challenge our insights on what the future Belgian energy system would look like if we are to reach net-zero GHG emissions by 2050. This, in turn, has allowed us to create a multitude of possible pathways towards 2050, all backed by Belgium’s most detailed techno-economic energy system model and rigorous, data-driven analysis.
Thus, it is at this intersection of science and real-world experience that we have tried to create a new landmark in data-driven analysis for a carbon neutral future Belgian energy system. And we are more than happy to share our key findings with you on this PATHS2050 Platform.
There are different roads that lead towards a carbon neutral future. And while they may differ in appearance, all of them share a need for thoughtful and decisive action.
Our insights on the possible pathways to a carbon neutral energy system by 2050 are based on data-driven analysis and modelling with the TIMES-Be model: a model which calculates different net-zero emissions scenarios based on the evolution of technical and economic parameters, and searches for the most cost-effective solution to meet the demand for energy services from today, all the way up to 2050.
A brief description of our modelling framework can be found here.
We are eager to present to you our key findings under three main pathways, also known as Scenarios
Key insights from the PATHS2050 Scenarios
All three modelled scenarios reduce CO2 emissions to nearly zero by 2050. This outcome is based on how the model was set up: it assumes net-zero CO2 emissions by 2050. Other greenhouse gases such as N2O, CH4 and F-gases are not included.
High CO₂ prices, assumed at 480 euro per ton by 2050, are effective in reducing emissions. However, without additional complementary policies, they are not sufficient to achieve net-zero in Belgium, with emissions reaching approximately 8–9 million tonnes per year. This conclusion is based on a near-zero sensitivity run.
Final energy demand and electrification
In all three scenarios, Belgium’s final energy demand (excluding feedstock) decreases by a third, from over 350 TWh in 2023 to about 250 TWh by 2050. This is mainly due to efficiency gains and electrification of end-user demands such as buildings (use of heat pumps) an road transport.
Electricity use more than doubles in all scenarios, from 80 TWh in 2025 to 155-170 TWh in 2050. Electrification is a no-regret measure, particularly for building heating and road transport. From 2030 onwards, industry offers the highest electrification potential, although this depends strongly on the availability of CO2 storage.
Growth in electricity demand between today and 2050 includes:
Road transport: +8 TWh by 2030, +14 TWh by 2050
Buildings (residential and commercial): +7 TWh by 2030, + 15 TWh by 2050
Industry: +6 TWh by 2030, +14 TWh by 2050 (potentially more if CCS is limited)
Data centres: from 2 TWh today to 12 TWh in 2050
Electricity generation and system needs
All scenarios require a significant increase in power generation and capacity to keep pace with rising electricity demand. Accelerated investments are needed to:
Expand offshore wind—both in the Belgian North Sea and far offshore—to nearly full potential is a no-regret option. Grid connections to the mainland and reinforcements within Belgium are essential and at least 3,5 GW offshore wind is made available by 2030.
Build out PV capacity at a steady, high pace, to at least 40 GW by 2050 (up from over 10 GW end of 2023)—averaging 1,2 GW per year. As a reference: in the record year 2023, Belgium added 1,8 GW of new PV capacity.
Firm capacity remains important. New gas turbines (CCGTs and CHPs) are required to accommodate the closure of nuclear capacity. These newer, more efficient turbines can support a net-zero 2050 future, running on synthetic gas, biomethane, or potentially hydrogen. Even in scenarios with flexible nuclear capacity, at least 7.5 GW fuel-based thermal capacity is needed by 2050, rising up to 9.3 GW depending on the scenario.
Belgium remains a net importer of electricity in all scenarios, with imports ranging from:
7 TWh/year in the ROTORS and REACTORS scenario
Over 30 TWh/year in the IMPORTS scenario
The role of Carbon Capture and Storage (CCS)
CCS will play a crucial role in reducing hard to decarbonise CO2 process emissions. It is not economically viable for power generation. Starting from 2030 with pilot projects, the model assumes CCS scales to 20 million tonnes per year and stays constant through 2050.
A scenario without CCS is feasible but would be more expensive and requires much higher imports of green molecules. In the ROTORS scenario, limited access to CCS combined with locally produced hydrogen enables domestic e-molecule production.
Methane and biomethane in the energy system
Methane remains important in a net-zero system. Today, natural gas—of wich methane is the largest component—makes up over 100 TWh of the final energy demand. By 2050, this drops to 21-35 TWh, depending on the scenario. In the scenarios with unlimited access to CCS, more natural gas remains in the energy system.
Key trends include:
Phase-out in low-temperature heating in buildings and industry
Continued use in high-temperature industrial processes
Peak natural gas use in power generation towards 2030, increasing from 42 to more than 50 TWh depending on the scenario. Towards 2050, gas turbines will be used as peak plants, with low operating hours.
Natural gas is gradually replaced by:
Imported e-methane (3-8 TWh), particularly for international aviation
Hydrogen derivatives
Biomethane and biomass (29-43 TWh combined, domestic and import)
Biomethane is mainly used in maritime transport for dual-fuel engines, helping to mitigate the higher cost of e-methane. Solid biomass is predominantly used in industry, and for electricity and heat production (CHPs). When combined with CCS in industry, this can lead to negative emissions, which would not be possible when biomass would be converted into biofuels and used in road transport or aviation.
Demand and supply of clean molecules
Clean molecules such as hydrogen, e-methane, e-methanol, ammonia and synthetic fuels will gain in importance in a net−zero emission future. Their role in the final energy demand remains very limited due to cost, with electrification almost always the better option.
However, EU legislation (e.g. RED III, FuelEU, ReFuelEU, ETS) will push their use in:
International aviation (70% sustainable fuels by 2050)
Maritime transport (net-zero by 2050)
That way, international transport will account for at least 57 TWh in all scenarios.
Apart from international transport, clean molecules are also needed in:
Remaining refinery operations
Industrial feedstocks
Power generation: 4 TWh (ROTORS/REACTORS), up to 20 TWh (IMPORTS)
The supply of clean molecules depends heavily on import. By 2050, the high need for clean international transport fuels such as e-methane, ammonia and e-kerosene will push the import of clean molecules to at least 69 TWh, up to more than 100 TWh in the IMPORTS scenario with lower costs for clean molecules.
Domestic hydrogen production
Domestic green hydrogen production from electrolysers linked to renewable production will be negligible. Exceptions include:
E-hydrocarbon production can become economically viable when having access to large quantities of affordable electricity while having limited CCS storage potential (ROTORS)
Availability of nuclear SMRs enables high-efficiency hydrogen production via Solid Oxide Electrolysis (SOE): 7-16 TWh by 2050
Hydrogen as a by-product of refineries and chlorine production: up to 6 TWh
Investment needs and system costs
Large-scale domestic low-carbon electricity production helps reduce long-term system costs, though it requires significant upfront investment. Total system costs include capital and operational expenditures, alongside evolving fuel costs. Savings are driven by a sharp decline in fossil fuel imports.
The ROTORS and REACTORS scenarios show similar total system costs. Differences between them fall within the margin of uncertainty for technology costs. Rather than debating small cost variations, the focus should be on ensuring electricity supply and implementing a robust long-term strategy. Between 2040 and 2050, these scenarios require annual investments of around 12.5 billion euro in the electricity sector.
Even in the IMPORTS scenario, total system costs are also in the same range. Lower costs for imported molecules help compensate for higher electricity import costs. By 2050, total costs in the IMPORTS scenario are around 3 billion euro lower. However, this difference is smaller than the potential impact of price shocks.
If molecule prices in the IMPORTS scenario turn out to be similar to those in the ROTORS or REACTORS scenarios, total annual costs could increase by 8.6 billion euro by 2050. In that case, IMPORTS becomes the most expensive scenario. Although it requires lower domestic investments, higher imports of molecules and electricity make Belgium more susceptible to price volatility.