Power Sector
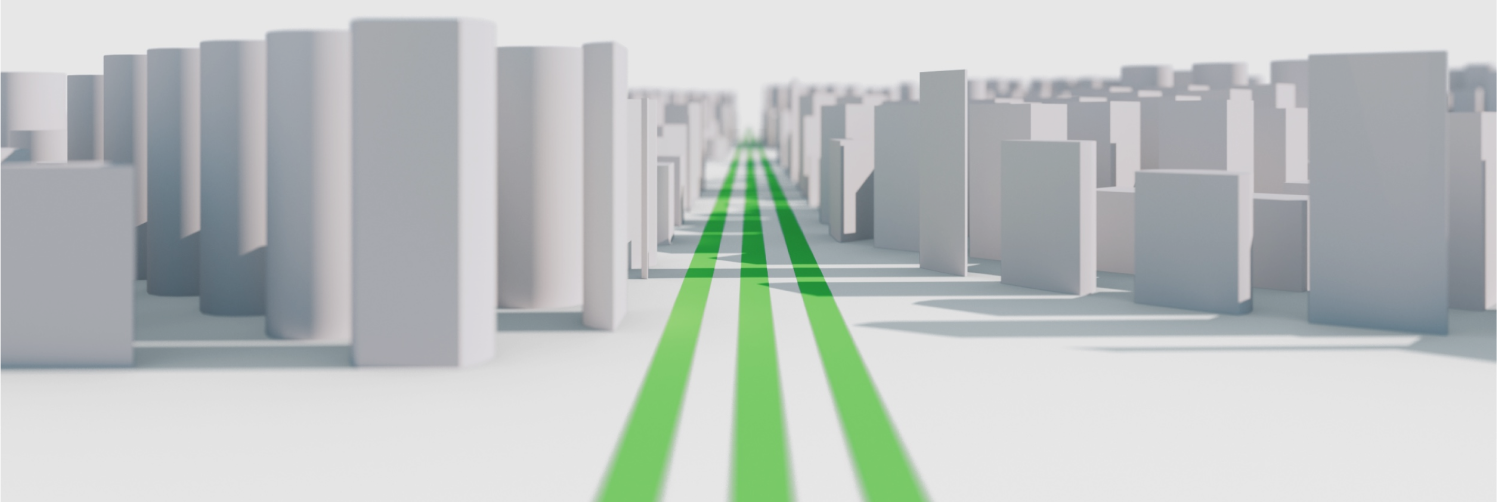
Electricity generation technology
Capacity
To support the transition to a carbon-neutral energy system in Belgium by 2050, both electricity generation and storage capacities need to at least double compared to 2025 levels. Starting from around 33 GW in 2025, total installed capacity grows to 98 GW in the ROTORS scenario, 92 GW in REACTORS, and 94 GW in IMPORTS.
Solar photovoltaic (PV) capacity grows substantially—by a factor of 2.6 to 3.2 from 2025 to 2050. Onshore wind capacity increases from 4 GW to 10 GW, and up to 17 GW in Rotors. Offshore wind capacity expands from the current 2,5 GW to 23 GW in ROTORS, and due to the more limited access to far offshore to 13 GW in the other two scenarios.
Fossil fuel capacity in 2050 is reduced by 15–45%, and remaining installations are converted to hybrid systems capable of using clean molecules. Nuclear capacity significantly expands where allowed: doubling to 4 GW in the ROTORS scenario and quadrupling to 8 GW in REACTORS. Biomass capacity doubles by 2050 in the ROTORS and IMPORTS scenarios but remains modest compared to other sources. Transmission and storage capacities are essential in all scenarios, with the IMPORTS scenario - logically - showing the most investment in these areas. By 2050 the model invests in 6-7.3 GW of battery storage, while vehicle-to-grid services provide 2,5 GW of flexibility to the system. Together with the pumped hydro storage this leads to at least 10 GW of storage capacity.
Each scenario reflects a different emphasis:
ROTORS features the highest levels of both onshore and offshore wind capacity. It has the lowest levels of PV, fossil/hybrid, and storage capacities. Biomass and geothermal energy play a stronger role, while nuclear is developed up to the scenario’s permitted limit. Here, intermittent wind generation is balanced by a mix of nuclear, biomass, geothermal, and storage.
REACTORS is defined by the highest nuclear and storage capacities. Biomass is minimized by design, while geothermal is still significant. Fossil/hybrid capacity falls between the levels seen in Rotors and Imports. Onshore wind is maximized, and offshore wind approaches the scenario limit. In this scenario, nuclear generation works in tandem with solar and storage to meet demand.
IMPORTS focuses on fossil/hybrid systems, transmission, and solar PV—each at the highest levels among the three scenarios. Biomass and geothermal are also well-developed. By design, there is no nuclear power in this scenario by 2050. Wind power is built to near-scenario limits, both onshore and offshore. With no nuclear option and greater access to imported clean molecules, this scenario leans toward a fuel-driven energy system supported by higher PV deployment, storage, and transmission capacity.
Generation
To support the transition to a carbon-neutral energy system in Belgium by 2050, electricity generation will need to double compared to 2025 levels. Starting at 88 TWh in 2025, electricity generation (including imported electricity) grows to 210 TWh in the ROTORS scenario, 186 TWh in REACTORS, and 171 TWh in IMPORTS. Belgium remains a net importer of electricity, with up to 6 TWh annual import by 2050 in ROTORS and REACTORS, to more than 30 TWh in IMPORTS.
Solar photovoltaic (PV) generation nearly triples across all scenarios. Onshore wind generation rises from 8 TWh to 22 TWh in Reactors and Imports, and up to 34 TWh in Rotors. Offshore wind generation grows from 8 TWh to 94 TWh in Rotors, and slightly more than 50 TWh in REACTORS and IMPORTS.
Biomass-based electricity doubles by 2050 in the ROTORS and IMPORTS scenarios, overtaking fossil-based generation. Fossil fuel generation sees a sharp decline—reduced by 75% to 90%—and is largely replaced by hybrid systems that can run on clean molecules. Nuclear generation increases significantly when permitted: doubling in the ROTORS scenario and quadrupling in REACTORS by 2050.
Each scenario showcases a distinct energy mix:
ROTORS leads in both onshore and offshore wind generation. It has the lowest reliance on PV and imports, while biomass and geothermal play a larger role. Nuclear energy is maximized up to the scenario’s limit. This mix of intermittent wind generation is balanced with stable supply from nuclear, biomass, and geothermal sources.
REACTORS is defined by the highest level of nuclear generation, with the lowest use of biomass and fossil/hybrid systems. PV generation is moderate—between the other two scenarios. Thanks to the strong nuclear electricity generation, the need for other dispatchable generation is significantly reduced.
IMPORTS shows the highest levels of fossil/hybrid, PV generation, and import. Biomass and geothermal sources are also heavily used. Nuclear energy is completely absent by 2050, as ruled out by scenario design. Both onshore and offshore wind are developed close to their respective scenario limits. The result is a fuel-driven energy system, reliant on clean molecule imports, complemented by high PV output, storage, and robust grid infrastructure.
KEY TAKEAWAYS
-
Up to 2030, new PV installment rate should be at least
1 GW per year
to reach a capacity of 17-19 GW
-
By 2030, offshore wind capacity should increase to
7-9,3 GW
In Belgian territorial waters and with direct access to far offshore
-
From 2040 earliest, new nuclear can become part of the energy system
4-8 GW
As large-scale EPR or more flexible SMR reactors
-
By 2050, batteries and vehicle-to-grid offer
8,5-10 GW
Of storage and flexibility to the electricity system
Electricity generation and demand profiles
Electricity generation traditionally follows demand. When a country’s electricity mix is dominated by controllable thermal power plants—known as dispatchable generation—supply can be planned and adjusted as needed. But the energy system is evolving. With the growing share of variable renewable sources like wind and solar, generation is increasingly influenced by the weather. As a result, weather forecasting becomes critical, and new markets emerge for short-term storage (like batteries) and flexible demand that can adapt to the available supply.
To better understand how this shift affects the system, we need to look beyond yearly averages and zoom in on hourly generation and demand patterns. That’s why EnergyVille developed a method based on 10 representative days spread throughout the year, divided into two-hour time blocks. This approach strikes a balance between capturing enough temporal detail—similar to more complex dispatch models—while keeping the analysis time manageable.
In the graphs below, we show the electricity generation and demand profile for a typical summer and winter day in 2050.
Summer
Generation profile typical summer day 2050
A typical summer day in 2050 is marked by high levels of solar power, with photovoltaic (PV) electricity production peaking during sunny hours. In addition, offshore wind farms—particularly those located far out at sea—generate a steady supply of electricity throughout the day.
However, there’s a clear distinction between different types of wind generation. Far-offshore wind performs strongly, while coastal offshore and onshore wind contribute only minimal amounts during summer. As a result, total wind electricity production is noticeably lower than in winter.
Fossil-based generation is barely used, if at all.
In scenarios that include nuclear energy (Rotors and Reactors), nuclear plants are active but adjusted depending on renewable availability. In the Reactors scenario, nuclear output is reduced during solar peaks. In the Rotors scenario, output is scaled down for most of the day due to higher wind generation.
Solar energy is also used strategically: during the day, it charges electric vehicles and fills up the pumped hydro reservoirs. Later, when the sun sets and PV output drops, stored battery energy is discharged to meet the remaining demand.
Across all three scenarios, electricity imports are used throughout the day to help balance the system. In the Rotors and Reactors scenarios, there’s also a notable amount of electricity exports, driven by surplus renewable generation.
Each scenario’s core design becomes evident on this summer day:
Rotors stands out with the highest share of far-offshore wind,
Reactors emphasizes nuclear energy, and
Imports relies most heavily on solar power.
Demand profile typical summer day 2050
On a typical summer day in 2050, electricity demand reaches its peak at nearly 25 GW in the Rotors scenario, 23 GW in the Reactors scenario, and 20 GW in the Imports scenario.
The industry sector is by far the largest consumer of electricity, followed by the commercial, transport, and residential sectors. Electricity use within the energy supply sector varies noticeably between scenarios—highest in Rotors—primarily due to differences in the production of clean molecules.
Electricity consumption remains relatively steady throughout the day across most sectors, with one major exception: transport. As planned, electric vehicle smart charging aligns with peak solar generation during sunny hours. It rises to around 7 GW, and at times even becomes negative when electric vehicle batteries feed power back into the grid.
Winter
Generation profile typical winter day 2050
On a typical winter day in 2050, electricity generation patterns look more balanced across all three scenarios compared to summer. The sharp rise and fall of solar power seen in summer isn’t present, resulting in a more stable daily profile.
Wind energy plays a bigger role in winter, with more consistent electricity generation across far-offshore, coastal offshore, and onshore locations. All wind types produce more power compared to a typical summer day.
Fossil-based generation is brought online to help balance supply and demand.
Nuclear power plants are active in the Rotors and Reactors scenarios. In Rotors, their output is steadier than in summer, though still occasionally reduced due to excess power. In Reactors, nuclear output fluctuates more significantly throughout the day.
Electricity imports and exports vary strongly between scenarios. The Imports scenario relies on steady imports throughout the day. Reactors sees only minimal imports, while Rotors, having direct access to far offshore wind, consistently 'exports' a significant amount of electricity.
Electricity storage is used the most in the Imports scenario—twice as much as in Reactors, with Rotors falling in between.
The core characteristics of each scenario are clear in the winter profile:
Rotors leads in offshore wind production,
Reactors shows the highest nuclear generation,
and Imports depends most on electricity imports and storage.
Demand profile typical winter day 2050
On a typical winter day, final electricity consumption peaks at around 29 TW in the Rotors scenario, 27 TW in the Reactors scenario, and 24 TW in the Imports scenario.
The industry sector is the largest consumer of electricity, followed by the residential, commercial, and transport sectors. Agriculture has relatively low demand. Electricity use in the supply sector—mainly for producing clean molecules—varies significantly between scenarios: highest in Rotors, lowest in Imports, with Reactors in between.
Electricity demand remains relatively stable throughout the day for most sectors, except for residential and transport.
Residential demand rises during the day and peaks in the evening, driven by heating needs.
Transport demand, largely from charging electric vehicles, follows the opposite pattern—peaking at night.
This contrasting behavior helps balance the grid: night-time EV charging complements daytime and evening heating demand in homes.
CO2 emissions power sector
By 2050, CO₂ emissions from electricity generation are (almost) entirely mitigated across all scenarios. Only in the Rotors scenario a small amount remains.
Back in 2025, power sector emissions still totalled 13 million tonnes (Mton). By 2030, emissions remain relatively stable in the Reactors and Imports scenarios, while the Rotors scenario already shows a modest decline.
The turning point comes in 2040, when all three scenarios begin to see significant emission reductions, with the Rotors scenario showing the sharpest drop due to the larger availability of offshore wind.
By 2050:
The Reactors and Imports scenarios achieve zero emissions from electricity generation.
The Rotors scenario is nearly there, with just 600 kilotonnes (kton) of residual emissions.
To ensure a level playing field across scenarios, the remaining 600 kton in Rotors is offset by reduced industrial emissions, aligning all scenarios with the same total remaining CO₂ emissions: 2 Mton.